In a recent article published in Nature, researchers developed a groundbreaking wireless digital bridge that helped a chronic tetraplegia patient to walk naturally on complex terrains.
More importantly, his neurological improvements persisted even after switching off the bridge.
Furthermore, this highly reliable brain-spine interface (BSI) calibrated within a few minutes remained stable over one year, even during independent use at home.
Study: Walking naturally after spinal cord injury using a brain–spine interface. Image Credit: ALPAPROD/Shutterstock.com
Background
Spinal cord injuries that disrupt the neurons in the lumbosacral spinal cord interrupt the brain-derived executive commands necessary to enable walking; consequently, a person suffers from severe (and permanent) paralysis.
About this study
In the present study, researchers carried out all experiments as part of the Stimulation Movement Overground (STIMO)-BSI, an ongoing clinical feasibility study aimed at functional assessment of the cortical devices before implantation.
A single-participant study
The team tested and validated this digital bridge in a 38-year-old male who sustained an incomplete cervical spinal cord injury ten years prior .
In the STIMO clinical trial, a five-month neurorehabilitation program helped him regain the ability to step forward with the aid of a front-wheel walker via targeted epidural electrical stimulation of the spinal cord.
Despite using stimulation at home for nearly three years, his neurological recovery plateaued, thus, he enrolled in STIMO-BSI.
Digital bridge, its neurosurgical implantation, and calibration
This BSI comprised fully implanted recording and stimulation systems that established a direct link between cortical activity and the analog modulation of epidural electrical stimulation programs that tune lower limb muscle activation to help regain standing and walking after paralysis due to a spinal cord injury.
Further, they planned pre-operative procedures to optimally position the BSI implants over the spinal cord and brain.
To this end, first, the researchers used computerized tomography (CT) and magnetoencephalography (MEG) to acquire functional and anatomical imaging data that helped them identify the cerebral cortex regions that responded vigorously to the intention to move both lower limbs.
Next, they uploaded the location of both implants onto a neuronavigation system. They discharged the participant 24 h after each neurosurgical intervention. A weighted Aksenova/Markov-switching multilinear algorithm calibrated a BSI in the first session after the neurosurgical intervention.
Its gating model computed the probability of the intention to move a joint and another multilinear model predicted the scale and directionality of the intended movement.
It enabled the participant to achieve a fivefold increase in hip flexor muscle activity within five minutes of calibration to generate torque with an accuracy of 97% compared to attempts without the BSI.
This BSI framework eventually enabled the participant to control seven states. The participant gradually controlled the movement of each joint bilaterally with an accuracy of 74±7%, and decoder latency was as low as 1.1 seconds for the seven states.
Walking on complex terrains requires balanced and sequential muscle activation that support bodyweight, propel, and swing left and right lower limbs. In fact, the ability to adjust limb movements to overcome obstacles or climb ramps or stairs is necessary for day-to-day mobility.
With the BSI, the participant climbed up and down a steep ramp nearly two times faster than without the BSI.
During 40 sessions of neurorehabilitation, including physiotherapy sessions, the participant accomplished walking, balancing, and managing single-joint movements with BSI.
He exhibited enhanced standing and walking capacities translating to an increase in his WISCI II scores from six to 16 after STIMO-BSI participation.
The participant also showed marked improvements in all the conventional clinical assessments, e.g., the six-minute walk test, assessed by physiotherapists blinded to the study. The study team followed up with the participant for three years.
Conclusions
Though the researchers validated this digital bridge in a single individual, they believed it would likely benefit a wide range of individuals with severe paralysis due to injury at other spinal locations due to the following three observations.
First, they have validated the physiological principles governing epidural electrical stimulation of the spinal cord in all individuals with complete (or incomplete) injuries.
Second, they successfully developed methods for rapid and stable link calibration enabling the patient to self-operate the BSI at home. Third, this brain decoding framework has shown similar robustness and stability in two other tetraplegic patients.
Indeed, establishing a digital bridge between the brain and spinal cord marks the beginning of a new chapter in the domain of motor deficit treatments.
-
Lorach, H. et al. (2023) "Walking naturally after spinal cord injury using a brain–spine interface", Nature. doi: 10.1038/s41586-023-06094-5. https://www.nature.com/articles/s41586-023-06094-5
Posted in: Medical Science News | Medical Research News | Medical Condition News | Healthcare News
Tags: Brain, Chronic, Clinical Trial, Cortex, CT, Epidural, Imaging, Implants, Muscle, Neurons, Paralysis, Physiotherapy, Spinal Cord Injury, Spine, Tomography, Walking
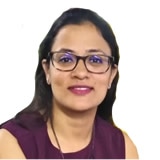
Written by
Neha Mathur
Neha is a digital marketing professional based in Gurugram, India. She has a Master’s degree from the University of Rajasthan with a specialization in Biotechnology in 2008. She has experience in pre-clinical research as part of her research project in The Department of Toxicology at the prestigious Central Drug Research Institute (CDRI), Lucknow, India. She also holds a certification in C++ programming.
Source: Read Full Article